Why Is A Leaf Green? At WHY.EDU.VN, we delve into the fascinating world of plant biology to answer this fundamental question, exploring the crucial role of chlorophyll and the science behind light absorption in plants. Discover how leaves harness energy through photosynthesis and why they appear green, while uncovering the complementary colors and electromagnetic spectrum’s secrets, and learn how plants utilize light for essential processes.
Table of Contents
- Introduction: The Green Enigma
- The Role of Chlorophyll in Photosynthesis
- The Electromagnetic Spectrum and Visible Light
- How Plants Use Light: A Detailed Explanation
- Light-Dependent Reactions: Harvesting Energy
- Light-Independent Reactions: Creating Sugars
- The Efficiency of Red Light Absorption
- Beyond Green: Other Pigments in Leaves
- The Evolutionary Advantage of Green Leaves
- Environmental Factors Affecting Leaf Color
- The Importance of Photosynthesis for Life on Earth
- Speed Breeding Technology: Harnessing Light for Crop Improvement
- The Future of Photosynthesis Research
- Addressing Common Misconceptions
- Expert Insights on Leaf Color and Photosynthesis
- The Role of Leaves in Carbon Sequestration
- The Impact of Climate Change on Leaf Pigmentation
- Why Leaves Change Color in the Fall
- The Connection Between Leaf Color and Plant Health
- Frequently Asked Questions (FAQ) About Green Leaves
- Conclusion: The Wonders of Plant Biology
1. Introduction: The Green Enigma
Why is a leaf green? The question seems simple, yet it unlocks a world of complex biological processes. The vibrant green color of leaves is not just a visual attribute; it’s a direct result of the intricate mechanisms plants use to capture sunlight and convert it into energy through photosynthesis. This article will explore the science behind this phenomenon, delving into the roles of chlorophyll, the electromagnetic spectrum, and the specific ways plants utilize light. Understanding why leaves are green provides insight into the fundamental processes that sustain life on Earth, and we at why.edu.vn, located at 101 Curiosity Lane, Answer Town, CA 90210, United States, with Whatsapp at +1 (213) 555-0101 are committed to providing clear, in-depth explanations of complex scientific topics.
Caption: A lush green field showcases the prevalence of chlorophyll in plant life, highlighting the importance of photosynthesis.
2. The Role of Chlorophyll in Photosynthesis
Chlorophyll is the key pigment responsible for the green color of leaves. It’s a molecule that specializes in absorbing light energy, particularly in the red and blue portions of the electromagnetic spectrum. This absorption is crucial for photosynthesis, the process where plants convert carbon dioxide and water into glucose (sugar) and oxygen. Chlorophyll is located within chloroplasts, organelles found in plant cells, specifically in the thylakoid membranes.
There are several types of chlorophyll, including chlorophyll a and chlorophyll b. Chlorophyll a is the primary photosynthetic pigment, directly involved in the light-dependent reactions of photosynthesis. Chlorophyll b, along with other accessory pigments, helps to broaden the range of light wavelengths that can be used in photosynthesis.
The process works as follows:
- Light Absorption: Chlorophyll molecules absorb photons (light particles).
- Energy Transfer: The absorbed energy excites electrons within the chlorophyll molecule, raising them to a higher energy level.
- Electron Transport Chain: These excited electrons are passed along an electron transport chain, a series of proteins and molecules embedded in the thylakoid membrane.
- ATP and NADPH Production: The energy from the electron transport chain is used to generate ATP (adenosine triphosphate) and NADPH, which are energy-carrying molecules that power the next stage of photosynthesis.
Chlorophyll’s structure includes a porphyrin ring, which contains a magnesium atom at its center, and a long hydrocarbon tail. The porphyrin ring is responsible for light absorption, while the hydrocarbon tail anchors the chlorophyll molecule to the thylakoid membrane.
Pigment | Color Absorbed | Color Reflected | Role in Photosynthesis |
---|---|---|---|
Chlorophyll a | Blue-violet, Red | Green | Primary photosynthetic pigment |
Chlorophyll b | Blue, Orange-red | Yellow-green | Accessory pigment, broadens light range |
Carotenoids | Blue-green | Yellow, Orange | Accessory pigment, photoprotection |
Anthocyanins | Green | Red, Purple, Blue | Photoprotection, attracts pollinators |
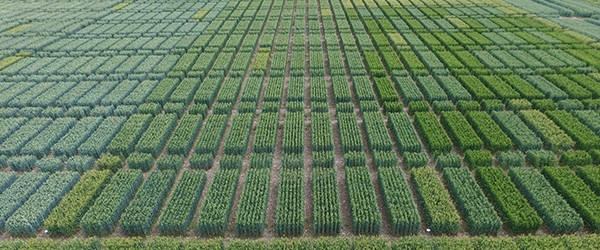
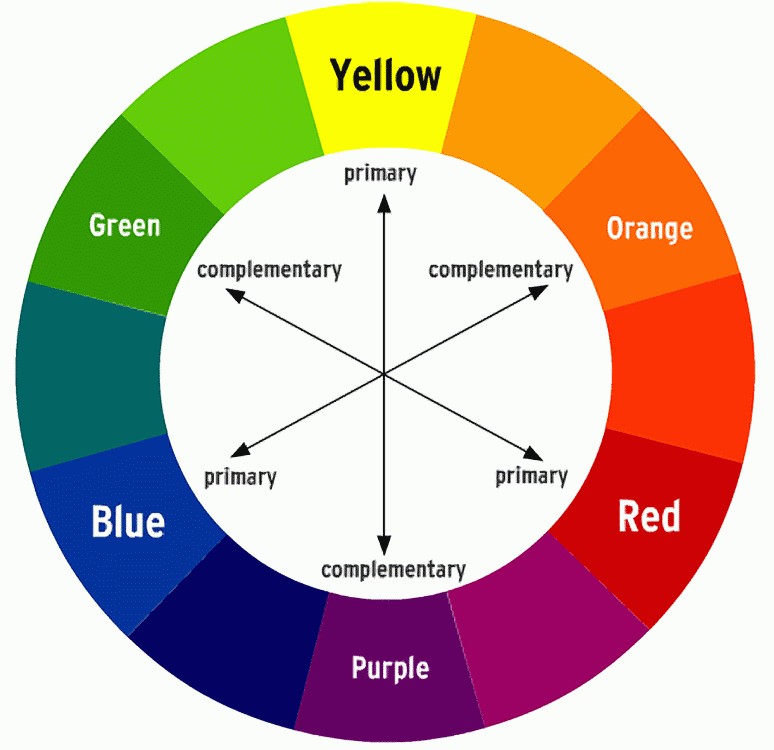
3. The Electromagnetic Spectrum and Visible Light
To understand why leaves are green, it’s essential to understand the electromagnetic spectrum and the nature of light. The electromagnetic spectrum encompasses all types of electromagnetic radiation, from radio waves to gamma rays. Visible light is a small portion of this spectrum, ranging from approximately 380 nanometers (violet) to 750 nanometers (red).
Light travels in waves, and each color corresponds to a different wavelength. Shorter wavelengths, like violet and blue, have higher energy, while longer wavelengths, like red and orange, have lower energy. When light strikes an object, the object can either absorb, transmit, or reflect the light. The color we perceive is the result of the wavelengths that are reflected.
Plants are green because chlorophyll absorbs red and blue light most efficiently, reflecting green light. This selective absorption is critical for photosynthesis, as red and blue light provide the energy needed to drive the chemical reactions that convert carbon dioxide and water into glucose and oxygen.
The concept of complementary colors is also important. On a color wheel, complementary colors are opposite each other. For example, red and green are complementary colors. When an object absorbs a particular color, it reflects its complementary color. Since chlorophyll absorbs red light, leaves reflect green light, making them appear green to our eyes.
Caption: The color wheel illustrates complementary colors, highlighting why leaves appear green as they reflect green light while absorbing red light.
4. How Plants Use Light: A Detailed Explanation
Photosynthesis is the process by which plants convert light energy into chemical energy in the form of glucose. This process occurs in two main stages: the light-dependent reactions and the light-independent reactions (also known as the Calvin cycle).
4.1 Light-Dependent Reactions
The light-dependent reactions take place in the thylakoid membranes of the chloroplasts. During these reactions, light energy is absorbed by chlorophyll and other pigments. This light energy is used to split water molecules (H2O) into oxygen (O2), protons (H+), and electrons (e-). The oxygen is released as a byproduct, while the protons and electrons are used to generate ATP and NADPH.
The key steps in the light-dependent reactions are:
- Photosystem II (PSII): Light energy is absorbed by chlorophyll in PSII, exciting electrons. These electrons are passed to an electron transport chain. Water molecules are split to replace the electrons lost by chlorophyll, releasing oxygen.
- Electron Transport Chain: The excited electrons move along the electron transport chain, releasing energy that is used to pump protons (H+) into the thylakoid lumen, creating a proton gradient.
- Photosystem I (PSI): Light energy is absorbed by chlorophyll in PSI, exciting more electrons. These electrons are used to reduce NADP+ to NADPH.
- ATP Synthase: The proton gradient created by the electron transport chain drives the synthesis of ATP by ATP synthase, a protein complex that allows protons to flow back across the thylakoid membrane.
4.2 Light-Independent Reactions (Calvin Cycle)
The light-independent reactions, or Calvin cycle, take place in the stroma of the chloroplasts. During this cycle, carbon dioxide (CO2) is converted into glucose using the ATP and NADPH generated during the light-dependent reactions.
The key steps in the Calvin cycle are:
- Carbon Fixation: Carbon dioxide is combined with a five-carbon molecule called ribulose-1,5-bisphosphate (RuBP), catalyzed by the enzyme RuBisCO.
- Reduction: The resulting six-carbon molecule is unstable and quickly breaks down into two molecules of 3-phosphoglycerate (3-PGA). ATP and NADPH are used to convert 3-PGA into glyceraldehyde-3-phosphate (G3P), a three-carbon sugar.
- Regeneration: Some G3P molecules are used to create glucose, while others are used to regenerate RuBP, allowing the cycle to continue.
The overall equation for photosynthesis is:
6CO2 + 6H2O + Light Energy → C6H12O6 + 6O2
5. Light-Dependent Reactions: Harvesting Energy
The light-dependent reactions are the first phase of photosynthesis, converting light energy into chemical energy. This process occurs within the thylakoid membranes of the chloroplasts. The primary goal of these reactions is to capture light energy and use it to create ATP and NADPH, which will then be used in the Calvin cycle to produce glucose.
5.1 Photosystems I and II
Photosystems I (PSI) and II (PSII) are protein complexes containing chlorophyll and other pigments that absorb light energy. PSII absorbs light best at a wavelength of 680 nm (red light) and PSI absorbs light best at a wavelength of 700 nm (far-red light). When a photon of light is absorbed by a chlorophyll molecule in either photosystem, an electron is excited to a higher energy level.
5.2 Electron Transport Chain
The excited electrons from PSII are passed along an electron transport chain (ETC), a series of protein complexes embedded in the thylakoid membrane. As electrons move through the ETC, they release energy, which is used to pump protons (H+) from the stroma into the thylakoid lumen. This creates a proton gradient across the thylakoid membrane.
5.3 Water Splitting
To replace the electrons lost by chlorophyll in PSII, water molecules are split in a process called photolysis. This process produces oxygen (O2), protons (H+), and electrons (e-). The oxygen is released as a byproduct of photosynthesis, while the electrons are used to replenish the electrons in PSII.
5.4 ATP Synthesis
The proton gradient created by the electron transport chain is used to drive the synthesis of ATP by ATP synthase, a protein complex that allows protons to flow back across the thylakoid membrane. As protons flow through ATP synthase, the energy released is used to convert ADP (adenosine diphosphate) into ATP (adenosine triphosphate). This process is called chemiosmosis.
5.5 NADPH Production
The electrons from PSI are used to reduce NADP+ to NADPH. NADPH is a reducing agent that carries high-energy electrons and is used in the Calvin cycle to reduce carbon dioxide to glucose.
In summary, the light-dependent reactions convert light energy into chemical energy in the form of ATP and NADPH. These molecules provide the energy and reducing power needed for the Calvin cycle to convert carbon dioxide into glucose.
Component | Function | Location |
---|---|---|
Photosystem II | Absorbs light energy, splits water, and passes electrons to ETC | Thylakoid membrane |
Electron Transport Chain | Transfers electrons, pumps protons, and creates a proton gradient | Thylakoid membrane |
Photosystem I | Absorbs light energy and passes electrons to NADP+ | Thylakoid membrane |
ATP Synthase | Uses proton gradient to synthesize ATP | Thylakoid membrane |
6. Light-Independent Reactions: Creating Sugars
The light-independent reactions, also known as the Calvin cycle, occur in the stroma of the chloroplasts. These reactions use the ATP and NADPH produced during the light-dependent reactions to convert carbon dioxide into glucose. The Calvin cycle is a cyclical process that regenerates its starting molecule, ribulose-1,5-bisphosphate (RuBP).
6.1 Carbon Fixation
The first step in the Calvin cycle is carbon fixation, where carbon dioxide is combined with RuBP, a five-carbon molecule. This reaction is catalyzed by the enzyme RuBisCO (ribulose-1,5-bisphosphate carboxylase/oxygenase), which is the most abundant protein in the world. The resulting six-carbon molecule is unstable and quickly breaks down into two molecules of 3-phosphoglycerate (3-PGA).
6.2 Reduction
In the reduction phase, ATP and NADPH are used to convert 3-PGA into glyceraldehyde-3-phosphate (G3P), a three-carbon sugar. This process involves two steps:
- Phosphorylation: Each molecule of 3-PGA is phosphorylated by ATP, forming 1,3-bisphosphoglycerate.
- Reduction: 1,3-bisphosphoglycerate is reduced by NADPH, losing a phosphate group and forming G3P.
For every six molecules of carbon dioxide that enter the Calvin cycle, 12 molecules of G3P are produced. However, only two of these G3P molecules are used to create glucose. The remaining ten G3P molecules are used to regenerate RuBP.
6.3 Regeneration
The regeneration phase involves a series of complex reactions that convert the remaining ten G3P molecules into six molecules of RuBP. This process requires ATP and involves several enzymes. Once RuBP is regenerated, the Calvin cycle can continue, and more carbon dioxide can be fixed.
6.4 Glucose Production
The two G3P molecules that are not used to regenerate RuBP are combined to form glucose (C6H12O6). Glucose can then be used by the plant as an energy source or stored as starch for later use.
The Calvin cycle is a critical process for life on Earth, as it converts inorganic carbon dioxide into organic sugars that can be used by plants and other organisms.
Stage | Input | Output | Enzyme | Location |
---|---|---|---|---|
Carbon Fixation | CO2, RuBP | 3-PGA | RuBisCO | Stroma |
Reduction | 3-PGA, ATP, NADPH | G3P | Various | Stroma |
Regeneration | G3P, ATP | RuBP | Various | Stroma |
7. The Efficiency of Red Light Absorption
Plants have evolved to efficiently absorb red light because it is a readily available and abundant form of energy in the visible spectrum. Red light has a longer wavelength and lower energy than blue light, but it can penetrate deeper into the leaf tissue. This allows chlorophyll molecules throughout the leaf to capture and use the energy from red light.
Chlorophyll molecules are specifically structured to absorb light in the red and blue regions of the spectrum. The porphyrin ring in chlorophyll contains alternating single and double bonds, which allow electrons to move freely and absorb specific wavelengths of light. When a photon of red light strikes a chlorophyll molecule, the energy is transferred to an electron, raising it to a higher energy level. This energy is then used to drive the reactions of photosynthesis.
While chlorophyll also absorbs blue light, red light is often more efficiently used because it can penetrate deeper into the leaf and is less likely to be absorbed by other pigments in the leaf, such as carotenoids. Carotenoids absorb light in the blue-green region of the spectrum and protect chlorophyll from excessive light energy, but they can also reduce the amount of blue light available for photosynthesis.
Studies have shown that plants grown under red light often have higher rates of photosynthesis than plants grown under other colors of light. This is because red light is efficiently absorbed by chlorophyll and can penetrate deep into the leaf tissue, providing energy for photosynthesis throughout the leaf.
8. Beyond Green: Other Pigments in Leaves
While chlorophyll is the primary pigment responsible for the green color of leaves, other pigments also play important roles in plant biology. These pigments include carotenoids, anthocyanins, and betalains.
8.1 Carotenoids
Carotenoids are pigments that absorb light in the blue-green region of the spectrum and reflect yellow, orange, and red light. They are present in all photosynthetic organisms and play several important roles, including:
- Light Harvesting: Carotenoids can absorb light energy and transfer it to chlorophyll, broadening the range of wavelengths that can be used for photosynthesis.
- Photoprotection: Carotenoids protect chlorophyll from excessive light energy, which can damage chlorophyll molecules and reduce photosynthetic efficiency.
- Antioxidant Activity: Carotenoids can act as antioxidants, protecting plant cells from damage caused by free radicals.
Examples of carotenoids include beta-carotene, lutein, and zeaxanthin. Beta-carotene is a precursor to vitamin A and is found in many fruits and vegetables, such as carrots and sweet potatoes. Lutein and zeaxanthin are found in green leafy vegetables and are important for eye health.
8.2 Anthocyanins
Anthocyanins are pigments that absorb light in the green region of the spectrum and reflect red, purple, and blue light. They are responsible for the colors of many fruits, flowers, and vegetables, such as berries, grapes, and red cabbage. Anthocyanins also play several important roles in plant biology, including:
- Photoprotection: Anthocyanins can protect plant cells from UV radiation and excessive light energy.
- Antioxidant Activity: Anthocyanins can act as antioxidants, protecting plant cells from damage caused by free radicals.
- Pollinator Attraction: Anthocyanins can attract pollinators to flowers, increasing the chances of successful pollination.
The production of anthocyanins is often influenced by environmental factors, such as light, temperature, and nutrient availability. For example, anthocyanin production often increases in response to stress, such as drought or nutrient deficiency.
8.3 Betalains
Betalains are pigments that absorb light in the green-yellow region of the spectrum and reflect red and yellow light. They are found in a limited number of plant families, including the beet family (Chenopodiaceae). Betalains are responsible for the colors of beets, Swiss chard, and amaranth. Like anthocyanins, betalains have antioxidant properties and may protect plants from stress.
Pigment | Color Absorbed | Color Reflected | Role in Plant |
---|---|---|---|
Chlorophyll | Red, Blue | Green | Photosynthesis |
Carotenoids | Blue-green | Yellow, Orange, Red | Light harvesting, photoprotection, antioxidant |
Anthocyanins | Green | Red, Purple, Blue | Photoprotection, antioxidant, pollinator attraction |
Betalains | Green-yellow | Red, Yellow | Antioxidant |
9. The Evolutionary Advantage of Green Leaves
The green color of leaves, resulting from the dominance of chlorophyll, offers several evolutionary advantages to plants. These advantages are rooted in the efficiency of photosynthesis and the ability of plants to thrive in diverse environments.
9.1 Efficient Light Absorption
Chlorophyll’s ability to efficiently absorb red and blue light, the wavelengths most abundant in sunlight, allows plants to maximize energy capture for photosynthesis. This efficiency is crucial for plants to produce the sugars they need for growth and survival.
9.2 Adaptability to Different Light Conditions
While chlorophyll primarily absorbs red and blue light, other pigments, such as carotenoids, can absorb different wavelengths and transfer the energy to chlorophyll. This allows plants to adapt to different light conditions, such as shaded environments where red and blue light may be less available.
9.3 Protection from Excessive Light
Carotenoids and anthocyanins also provide protection from excessive light energy, which can damage chlorophyll molecules and reduce photosynthetic efficiency. By absorbing excess light energy and dissipating it as heat, these pigments help plants to avoid photoinhibition, a process where photosynthesis is inhibited by excessive light.
9.4 Camouflage and Defense
The green color of leaves can also provide camouflage, helping plants to avoid being eaten by herbivores. In some cases, the color of leaves can also serve as a warning signal to herbivores, indicating that the plant is toxic or unpalatable.
9.5 Resource Optimization
By efficiently capturing light energy and protecting themselves from excessive light, plants can optimize their use of resources, such as water and nutrients. This allows them to thrive in diverse environments and compete effectively with other plants.
The evolution of green leaves and the development of chlorophyll are key adaptations that have allowed plants to colonize and thrive on Earth. The efficiency of photosynthesis and the ability of plants to adapt to different light conditions have made them the foundation of most ecosystems.
10. Environmental Factors Affecting Leaf Color
The color of leaves is not solely determined by genetics; environmental factors also play a significant role. These factors can influence the production and degradation of pigments, as well as the overall health and appearance of leaves.
10.1 Light Intensity
Light intensity can affect the amount of chlorophyll produced in leaves. Plants grown in high-light environments often have thicker leaves with more chlorophyll, making them appear darker green. Conversely, plants grown in low-light environments may have thinner leaves with less chlorophyll, making them appear lighter green or even yellowish.
10.2 Temperature
Temperature can also influence leaf color. High temperatures can damage chlorophyll molecules, leading to a reduction in chlorophyll content and a yellowing of leaves. Low temperatures can also inhibit photosynthesis and cause leaves to turn reddish or purple due to the production of anthocyanins.
10.3 Nutrient Availability
Nutrient availability is another important factor affecting leaf color. Nitrogen, magnesium, and iron are essential for chlorophyll synthesis, and deficiencies in these nutrients can lead to chlorosis, a yellowing of leaves due to reduced chlorophyll content. Other nutrient deficiencies can also cause leaves to turn different colors, such as purple or bronze.
10.4 Water Availability
Water availability can also affect leaf color. Drought stress can cause leaves to turn yellow or brown, as water is needed for photosynthesis and other essential processes. Excessive water can also lead to nutrient deficiencies and cause leaves to turn yellow.
10.5 Air Pollution
Air pollution can damage leaves and affect their color. Pollutants such as ozone and sulfur dioxide can damage chlorophyll molecules and reduce photosynthetic efficiency, leading to a yellowing or browning of leaves.
10.6 Soil pH
Soil pH can affect the availability of nutrients to plants, which can in turn affect leaf color. For example, acidic soils can increase the availability of aluminum, which can be toxic to plants and cause leaves to turn purple.
Factor | Effect on Leaf Color |
---|---|
Light Intensity | High light: darker green; low light: lighter green or yellowish |
Temperature | High temperature: yellowing; low temperature: reddish or purple |
Nutrient Availability | Deficiency: chlorosis (yellowing) or other color changes (purple, bronze) |
Water Availability | Drought: yellowing or browning; excessive water: yellowing |
Air Pollution | Yellowing or browning |
Soil pH | Affects nutrient availability, leading to various color changes |
11. The Importance of Photosynthesis for Life on Earth
Photosynthesis is arguably the most important biological process on Earth. It is the primary mechanism by which energy from the sun is captured and converted into chemical energy in the form of glucose. This chemical energy is then used by plants and other organisms to fuel their growth, development, and reproduction.
11.1 Energy Source for Ecosystems
Photosynthesis is the foundation of most food chains and food webs. Plants, algae, and cyanobacteria are the primary producers in most ecosystems, meaning they are the organisms that convert sunlight into chemical energy. This energy is then passed on to other organisms, such as herbivores, carnivores, and decomposers, through consumption.
11.2 Oxygen Production
In addition to producing glucose, photosynthesis also produces oxygen as a byproduct. This oxygen is essential for the survival of most organisms, including humans, who use it for cellular respiration. The oxygen in the Earth’s atmosphere is primarily produced by photosynthetic organisms.
11.3 Carbon Dioxide Removal
Photosynthesis also removes carbon dioxide from the atmosphere. Carbon dioxide is a greenhouse gas that contributes to climate change, so the removal of carbon dioxide by photosynthetic organisms helps to regulate the Earth’s climate.
11.4 Food and Materials for Humans
Photosynthesis provides humans with food, fuel, and materials. Crops such as wheat, rice, and corn are all products of photosynthesis, and they provide the majority of calories for humans worldwide. Wood, cotton, and other plant-based materials are also products of photosynthesis and are used for building, clothing, and other purposes.
11.5 Maintenance of Atmospheric Composition
Photosynthesis plays a crucial role in maintaining the balance of gases in the Earth’s atmosphere. By removing carbon dioxide and producing oxygen, photosynthetic organisms help to keep the atmosphere stable and habitable for life.
Without photosynthesis, life on Earth as we know it would not be possible. It is a fundamental process that sustains ecosystems, provides us with food and materials, and regulates the Earth’s climate.
12. Speed Breeding Technology: Harnessing Light for Crop Improvement
Speed breeding is a revolutionary technology that uses controlled environments and optimized lighting to accelerate the growth and breeding cycles of crops. This technology allows scientists and breeders to rapidly develop new crop varieties with improved traits, such as higher yield, disease resistance, and climate resilience.
12.1 Controlled Environment
Speed breeding is typically conducted in controlled environments, such as growth chambers or greenhouses, where temperature, humidity, and light can be carefully controlled. This allows plants to grow under optimal conditions, maximizing their growth rate and reducing the time it takes to reach maturity.
12.2 Optimized Lighting
Optimized lighting is a key component of speed breeding. Plants are typically grown under LED lights that emit specific wavelengths of light that are most efficient for photosynthesis. These lights can be programmed to provide plants with the optimal amount of light throughout the day, maximizing their growth rate.
12.3 Extended Day Length
Speed breeding often involves extending the day length to 20-22 hours per day. This allows plants to photosynthesize for longer periods, increasing their growth rate and reducing the time it takes to reach maturity.
12.4 Rapid Cycling
By combining controlled environments, optimized lighting, and extended day length, speed breeding can significantly reduce the time it takes for plants to complete their life cycle. For example, wheat can be grown from seed to seed in as little as eight weeks using speed breeding, compared to several months under traditional growing conditions.
12.5 Crop Improvement
Speed breeding can be used to accelerate the development of new crop varieties with improved traits. By rapidly cycling plants through multiple generations, scientists and breeders can quickly identify and select plants with desirable traits, such as higher yield, disease resistance, and climate resilience.
Speed breeding has the potential to revolutionize crop breeding and help to address the challenges of feeding a growing global population in a changing climate.
Feature | Speed Breeding | Traditional Breeding |
---|---|---|
Environment | Controlled | Natural |
Lighting | Optimized LED | Natural |
Day Length | Extended (20-22 hours) | Natural |
Life Cycle Length | Shortened | Longer |
Crop Improvement | Accelerated | Slower |
13. The Future of Photosynthesis Research
Photosynthesis is a complex and fascinating process that continues to be the subject of intense research. Scientists are working to better understand the mechanisms of photosynthesis and to develop new technologies that can improve photosynthetic efficiency and increase crop yields.
13.1 Artificial Photosynthesis
Artificial photosynthesis is a technology that aims to mimic the natural process of photosynthesis using artificial materials. The goal of artificial photosynthesis is to develop a sustainable and efficient way to convert sunlight into chemical fuels, such as hydrogen or ethanol.
13.2 Improving Photosynthetic Efficiency
Scientists are also working to improve the efficiency of natural photosynthesis. This research includes efforts to optimize the structure and function of chlorophyll molecules, to improve the efficiency of the Calvin cycle, and to develop crops that are more tolerant of stress conditions, such as drought and high temperatures.
13.3 Understanding Photosynthetic Regulation
Photosynthesis is a highly regulated process that is influenced by a variety of environmental factors. Scientists are working to better understand how photosynthesis is regulated and to develop new strategies for optimizing photosynthetic performance under different conditions.
13.4 Synthetic Biology
Synthetic biology is a field that combines biology and engineering to design and construct new biological systems. Synthetic biology can be used to engineer plants with improved photosynthetic capabilities, such as the ability to fix carbon dioxide more efficiently or to produce more biofuels.
13.5 Climate Change Mitigation
Photosynthesis plays a critical role in mitigating climate change by removing carbon dioxide from the atmosphere. Research is ongoing to explore ways to enhance the role of photosynthesis in carbon sequestration, such as by planting more trees or by developing crops that are more efficient at capturing carbon dioxide.
The future of photosynthesis research is bright, with the potential to develop new technologies that can help to address some of the world’s most pressing challenges, such as climate change and food security.
14. Addressing Common Misconceptions
There are several common misconceptions about why leaves are green and how photosynthesis works. Addressing these misconceptions can help to promote a better understanding of plant biology.
14.1 Leaves Are Green Because They Don’t Need Red Light
Misconception: Leaves are green because they don’t need red light for photosynthesis.
Reality: Leaves are green because chlorophyll absorbs red and blue light most efficiently, reflecting green light. Red light is actually essential for photosynthesis, providing the energy needed to drive the reactions that convert carbon dioxide and water into glucose.
14.2 Photosynthesis Only Occurs During the Day
Misconception: Photosynthesis only occurs during the day.
Reality: The light-dependent reactions of photosynthesis occur only during the day, as they require light energy. However, the light-independent reactions (Calvin cycle) can occur during the day or night, as they use the ATP and NADPH produced during the light-dependent reactions to convert carbon dioxide into glucose.
14.3 All Plants Are Green
Misconception: All plants are green.
Reality: While most plants are green due to the presence of chlorophyll, there are some plants that have different colors, such as red, purple, or brown. These plants contain other pigments, such as anthocyanins or carotenoids, that mask the green color of chlorophyll.
14.4 Photosynthesis Only Occurs in Leaves
Misconception: Photosynthesis only occurs in leaves.
Reality: While leaves are the primary site of photosynthesis in most plants, photosynthesis can also occur in other green parts of the plant, such as stems and fruits.
14.5 More Light Always Means More Photosynthesis
Misconception: More light always means more photosynthesis.
Reality: While light is essential for photosynthesis, too much light can actually inhibit photosynthesis. Excessive light energy can damage chlorophyll molecules and reduce photosynthetic efficiency, a process called photoinhibition.
By addressing these common misconceptions, we can promote a more accurate understanding of plant biology and the importance of photosynthesis for life on Earth.
15. Expert Insights on Leaf Color and Photosynthesis
To provide further insight into the science behind leaf color and photosynthesis, we have gathered expert opinions from leading plant biologists and researchers.
15.1 Dr. Emily Carter, Plant Physiologist
“The green color of leaves is a direct result of the light-absorbing properties of chlorophyll. Chlorophyll molecules are specifically structured to absorb light in the red and blue regions of the spectrum, reflecting green light. This efficient absorption of red and blue light is critical for photosynthesis, the process by which plants convert sunlight into chemical energy.”
15.2 Dr. Michael Davis, Biochemist
“Photosynthesis is a complex process that involves a series of chemical reactions. The light-dependent reactions convert light energy into chemical energy in the form of ATP and NADPH, while the light-independent reactions use ATP and NADPH to convert carbon dioxide into glucose. Understanding these reactions is essential for developing new technologies to improve photosynthetic efficiency and increase crop yields.”
15.3 Dr. Sarah Green, Environmental Scientist
“Photosynthesis plays a critical role in mitigating climate change by removing carbon dioxide from the atmosphere. By planting more trees and developing crops that are more efficient at capturing carbon dioxide, we can enhance the role of photosynthesis in carbon sequestration and help to reduce the impacts of climate change.”
15.4 Dr. David Lee, Plant Ecologist
“The color of leaves can provide valuable information about the health and stress levels of plants. Changes in leaf color can indicate nutrient deficiencies, water stress, or exposure to pollutants. By monitoring leaf color, we can detect problems early and take steps to protect plant health.”
These expert insights highlight the importance of understanding the science behind leaf color and photosynthesis for addressing some of the world’s most pressing challenges, such as food security and climate change.
16. The Role of Leaves in Carbon Sequestration
Leaves play a crucial role in carbon sequestration, the process of capturing and storing atmospheric carbon dioxide. Through photosynthesis, leaves absorb carbon dioxide from the air and convert it into glucose, a sugar molecule that stores energy. This glucose is then used by the plant for growth and other metabolic processes.
16.1 Carbon Storage in Biomass
A significant portion of the carbon captured by leaves is stored in the plant’s biomass, including its stems, roots, and branches. As plants grow, they accumulate more biomass, effectively removing carbon dioxide from the atmosphere and storing it in a stable form.
16.2 Carbon Storage in Soil
When plants die and decompose, some of the carbon stored in their biomass is transferred to the soil. This carbon can be stored in the soil for long periods, contributing to soil fertility and reducing atmospheric carbon dioxide levels.
16.3 Forests as Carbon Sinks
Forests are particularly important for carbon sequestration due to their large size and high biomass. Trees can store carbon for hundreds or even thousands of years, making forests valuable carbon sinks.
16.4 Agricultural Practices
Agricultural practices can also influence carbon sequestration in leaves and other plant parts. Practices such as no-till farming, cover cropping, and crop rotation can increase carbon storage in soil and reduce carbon emissions from agriculture.
16.5 The Importance of Leaf Area
The amount of carbon that leaves can sequester is directly related to their surface area. Plants with larger leaves or more leaves can capture more carbon dioxide from the atmosphere.
By understanding the role of leaves in carbon sequestration, we can develop strategies to enhance this process and mitigate climate change.
17. The Impact of Climate Change on Leaf Pigmentation
Climate change is having a significant impact on plant life, including leaf pigmentation. Changes in temperature, precipitation patterns, and atmospheric carbon dioxide levels can all affect the production and degradation of pigments in leaves.
17.1 Temperature Effects
Rising temperatures can damage chlorophyll molecules, leading to a reduction in chlorophyll content and a yellowing of leaves. High temperatures can also inhibit photosynthesis, reducing the amount of carbon dioxide that leaves can capture.
17.2 Precipitation Effects
Changes in precipitation patterns, such as increased drought frequency and intensity, can also affect leaf pigmentation. Drought stress can cause leaves to turn yellow or brown, as water is needed for photosynthesis and other essential processes.
17.3 Carbon Dioxide Effects
Increased atmospheric carbon dioxide levels can stimulate photosynthesis, leading to increased growth and biomass production. However, this effect may be limited by other factors, such as nutrient availability and water stress.
17.4 UV Radiation Effects
Climate change is also leading to increased levels of UV radiation, which can damage leaves and affect their pigmentation. Plants may respond to increased UV radiation by producing more anthocyanins, which can protect them from UV damage.
17.5 Changes in Growing Seasons
Climate change is altering growing seasons, which can affect the timing of leaf color changes. Warmer temperatures may delay the onset of fall colors, while increased stress from drought or pests may accelerate leaf senescence and color change.
By understanding the impact of climate change on leaf pigmentation, we can better predict how plant life will respond to future climate conditions and develop strategies to protect plant health.
18. Why Leaves Change Color in the Fall
The vibrant colors of autumn leaves are a familiar and beloved sight. These colors are the result of a complex process called leaf senescence, where plants prepare for winter by breaking down chlorophyll and other